Abstract
The synthesis of diosgenyl 2-amino-2-deoxy-β-D-galactopyranoside is presented for the first time. This synthetic saponin was transformed into its hydrochloride as well as N-acyl, 2-ureido, N-alkyl, and N,N-dialkyl derivatives. Antifungal and antibacterial studies show that some of the obtained compounds are active against Gram-positive bacteria and Candida type fungi.
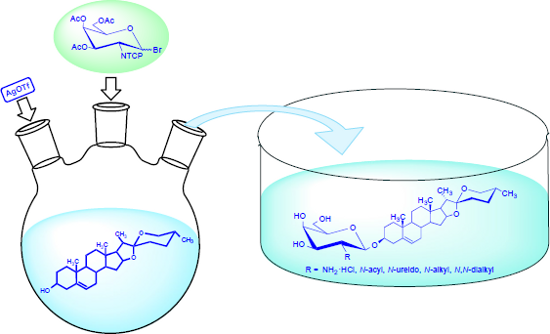
Graphical Abstract
Introduction
Saponins are steroid or triterpenoid glycosides found in various plants [1]. They produce a soap-like foam when shaken in aqueous solutions, which makes them useful as detergents, foaming agents and emulsifiers. Saponins are known for their structural diversity and a wide range of biological properties [2]. Among others, they display different pharmacological activities, particularly antifungal [3-6] and antitumor [7-9].
The aglycone part of a saponin is termed sapogenin. Diosgenin, yamogenin, tigogenin, smilagenin and sarsapogenin are the most abundant sapogenins in nature [10]. They are linked via a glycosidic bond to a sugar unit, mainly D-glucose. Diosgenyl glycosides constitute a very important group among spirostanol saponins. Diosgenin has a double bond between the C-5 and C-6 atoms of the spirostanol skeleton and can be found in combination with different sugars in Costus, Discorea, Paris, Solanum, Yucca, and Trillium plants [11]. The plants containing diosgenyl saponins are used in folk medicine in many countries. Such herbal medicines exhibit anti-inflammatory, antibacterial, antifungal, antiviral, diuretic and expectorant activities [2,11]. Importantly, these also help fight cancer cells [12-14].
Although saponins, in which D-galactose is directly bound with sapogenin are rarely found in plants, there are some examples of such galactosides. For example, timosaponin, isolated from an Anemarrhena asphodeloides plant, is a natural spirostanol saponin, which has D-galactose bound with sarsapogenin [15]. Tribulus plants are known to contain tigogenin linked with D-galactose [16]. Moreover Smilacina atropurpureea, Solanum indicum, and the genus Yucca contain smilacinosides and funkiosides [17], indiosides [18], and elephanosides [19]. All these saponins are glycosides, where diosgenin is attached directly with D-galactose.
Besides their isolation from natural sources, the chemical syntheses of saponins have been intensively investigated to evaluate and improve their pharmacological activities [20-22]. For example, in search of new variants of steroidal glycosides, diosgenyl 2-amino-2-deoxy-β-D-glucopyranoside was synthesized [23]. Modifications of the amino group in this synthetic saponin led to analogs with promising antitumor, antifungal and antibacterial activities [24-31].
In this paper, for the first time, syntheses of diosgenyl 2-amino-2-deoxy-β-D-galactopyranoside, its hydrochloride as well as N-acyl, 2-ureido, N-alkyl, and N,N-dialkyl derivatives are presented. These new synthetic saponins were tested for their antifungal and antibacterial activities.
Results and Discussion
Chemistry
Relying on our previous experiences [30,31], we used the O-acetylated bromide 2 to synthesize diosgenyl 2-amino-2-deoxy-β-D-galactopyranoside (4) (Scheme 1). This glycosyl donor was N-protected with the tetrachlorophthaloyl (TCP) group.
Scheme 1: Synthesis of diosgenyl 2-amino-2-deoxy-β-D-galactopyranoside (4).
Scheme 1: Synthesis of diosgenyl 2-amino-2-deoxy-β-D-galactopyranoside (4).
The synthesis of 4 started from commercially available D-galactosamine hydrochloride which was first converted to the 2-N-tetrachlorophthaloyl derivative followed by peracetylation with acetic anhydride to give fully protected galactose 1 as an anomeric mixture. The α and β anomers (3:7 molar ratio) were identified by NMR. To synthesize bromide 2, we used TiBr4 as a bromination agent. Bromide 2 was obtained as a mixture of α and β anomers (1:4 molar ratio) and was used directly in the glycosylation. This mixture of 2 is chromatographically inseparable due to the highly reactive nature of the bromine group at the anomeric carbon, but, the anomers of 2 are readily distinguishable in the NMR spectrum (δ 6.65, d, J1,2 = 3.7 Hz for the α anomer and δ 6.35, d, J1,2 = 9.6 Hz for the β anomer).
Glycosylation of diosgenin with 2 was performed in dichloromethane by a “reverse” procedure: The glycosyl donor was added to the solution of diosgenin and the promoter (silver triflate) [31]. This procedure afforded the expected β glycoside 3 in 80% yield. The structure of 3 was determined by 1H and 13C NMR supported by COSY and HSQC techniques. The β linkage in 3, as well as the 4C1 conformation of the pyranose ring were confirmed by the coupling constant of the anomeric proton (J1,2 = 8.4 Hz). The deprotection of 3 was achieved by using 98% hydrazine hydrate in refluxing ethanol. This procedure removes the TCP and acetyl protecting groups in a one-pot reaction and yields diosgenyl 2-amino-2-deoxy-β-D-galactopyranoside (4). Saponin 4 treated with the HCl in MeOH was converted into hydrochloride 5. To explore the influence of different modifications of the amino group in 4 on its antimicrobial activity the N-acyl (6, 7), 2-ureido (8–10), N-monoalkyl (11) and N,N-dialkyl (12, 13) derivatives of diosgenyl 2-amino-2-deoxy-β-D-galactopyranoside were also synthesized (Figure 1).
Figure 1: Derivatives of diosgenyl glycosides 5–13.
Figure 1: Derivatives of diosgenyl glycosides 5–13.
The N-acetyl derivative 6 was obtained by the treatment of 4 with acetic anhydride in methanol with Et3N whereas N-trifluoroacetyl derivative 7 was obtained in a reaction of 4 with trifluoroacetic anhydride in pyridine. The IR, HRMS and NMR confirmed the structures of 6 and 7. For example, the IR spectra of 6 and 7 show typical amide I and amide II bands at 1715–1650 cm−1. In turn, the 13C NMR spectrum of 7 is characterized by a quartet of the carbonyl carbon (≈157 ppm) with the JC,F coupling constant ≈39 Hz and a quartet of the CF3 carbon (≈117 ppm) with the JC,F coupling constant ≈290 Hz (see Supporting Information File 1 for experimental and NMR data).
Strategies for the preparation of ureido sugars usually involve the condensation of saccharides with ureas or the reaction of glycosylamines, amino sugar, or aminoglycosides with isocyanates, or their equivalents such as carbamates [32,33]. Ureido saponins presented here were obtained in the reaction of ethyl isocyanate (8), chloroethyl isocyanate (9) and phenyl isocyanate (10) with the amino group of 4. Isocyanate was added to the 1:1 (v/v) chloroform–methanol solution of 4 and Et3N each time. The yields of these reactions were very good (90% for 8) or good (60% for 9). However, phenylurea derivative 10 was isolated with only 34% yield, and the formation of various byproducts was observed in this case. The structures of saponins 8–10 were established using IR, HRMS and NMR spectra. For example, the amide I and amide II bands at 1665–1550 cm−1 are visible in the IR spectra and the carbonyl carbon signals at 160–170 ppm are present in the 13C NMR spectra of 8–10 (see Supporting Information File 1 for experimental and NMR data).
To obtain the N-alkyl derivatives of 4, a method called “two-step reductive alkylation of amines” was chosen. This method was successfully used to prepare N-alkyl derivatives of other amino sugars, including diosgenyl aminoglucosides [34,35]. The presented reductive alkylation consists of a reaction of amine 4 with the appropriate aldehyde, which results in the respective imine subsequently reduced with sodium cyanoborohydride to the alkylated amine. Thus, the reaction of 4 with 1.5 molar excess of acetaldehyde followed by the in situ reduction with NaBH3CN resulted in N-ethylamino (11) and N,N-diethylamino (12) saponins, which were separated by column chromatography. An analogous reaction of 4 with 3 molar excess of propionaldehyde, followed by the reduction with NaBH3CN resulted solely in N,N-dipropylaminosaponin 13. The 1H and 13C NMR spectroscopy as well as the HRMS spectrometry confirmed the structures of 11–13 (see Supporting Information File 1 for experimental and NMR data).
Evaluation of antimicrobial activity
The antimicrobial in vitro activities of 5–13 were tested against two fungal strains: Candida albicans and Candida tropicalis, and against the following six Gram-positive bacteria species: Enterococcus faecalis, Enterococcus faecium, Staphylococcus aureus, Staphylococcus epidermidis, Streptococcus pyogenes and Streptococcus pneumoniae. The minimum inhibitory concentrations (MICs) were determined by the broth microdilution method according to Clinical and Laboratory Standards Institute (CLSI) recommendations (for details see Supporting Information File 1). The MICs values are grouped in Table 1 and Table 2.
Table 2: Minimum inhibitory concentration (MIC) [μg/mL] for 5–13 against Gram-positive bacteria.
Compd. | E. faecalis | E. faecium | S. aureus ATCC 25923 | S. aureus ATCC 6538 | S. aureus ATCC 6538/P | S. epidermidis | S. pyogenes | S. pneumoniae |
5 | >1024 | >1024 | >1024 | >1024 | >1024 | >1024 | >1024 | >1024 |
6 | 16 | 8 | 16 | 32 | 32 | 8 | 8 | 32 |
7 | 32 | >1024 | >1024 | >1024 | >1024 | >1024 | >1024 | >1024 |
8 | 512 | 16 | >1024 | >1024 | >1024 | >1024 | >1024 | >1024 |
9 | 16 | 8 | 16 | 16 | 32 | 8 | 8 | 8 |
10 | >1024 | >1024 | >1024 | >1024 | >1024 | >1024 | >1024 | >1024 |
11 | 8 | 2 | 4 | 4 | 8 | 2 | 2 | 2 |
12 | 16 | >1024 | >1024 | 16 | 64 | 32 | 8 | 32 |
13 | 64 | >1024 | >1024 | 64 | 128 | 32 | 64 | 32 |
The presented results indicate that some of the tested saponins are characterized by selective activity. Thus, the hydrochloride of 5 inhibits the growth of the fungus genus Candida; however, this saponin is more active against C. tropicalis (MIC 4 μg/mL) than against C. albicans (MIC 64 μg/mL). In turn, hydrochloride 5 has no inhibitory activity against the tested Gram-positive bacteria. The N-trifluoroacetyl derivative of diosgenyl 2-amino-2-deoxy-β-D-galactopyranoside 7 is active solely against E. faecalis. In contrast to 7, the N-acetyl saponin 6 was found to inhibit the growth of all the tested fungi and bacteria with MICs of 8–32 μg/mL. Among the three ureido derivatives of 4, solely diosgenyl 2-(2-chloroethylureido)-2-deoxy-β-D-galactopyranoside (9) exhibits a good activity against all the tested pathogens with MICs of 8–64 µg/mL, whereas 8 was effective against C. tropicalis and E. faecium only and compound 10 does not exhibit any antimicrobial activity. Among the presented saponins, N-ethyl derivative 11 exhibits the best antimicrobial activity against all the tested microorganisms with MIC values of 2–8 µg/mL. Both N,N-diethyl (12) and N,N-dipropyl (13) derivatives are active against both fungal and almost all the bacterial strains except E. faecium PCM 1859 and S. aureus ATCC 25923. These two strains of bacteria are completely resistant to 12 and 13, whereas the growth of E. faecalis PCM 2673 as well as S. aureus ATCC 6538, and S. aureus ATCC 6538/P is inhibited by 12 and 13.
The analysis of the relationship between structure and biological activity of the presented derivatives of diosgenyl 2-amino-2-deoxy-β-D-galactopyranoside (4) shows that N-alkylation of the amine function is the most advantageous way of modification. An introduction of one ethyl (11), two ethyl (12) or two propyl (13) groups at the amine function improves the antifungal properties of saponins 11–13 in comparison with hydrochloride 5 and the remaining compounds. In the case of antibacterial properties, N-ethyl derivative 11 is the most active among the all tested compounds. Moreover, derivative 11 acts against all tested Gram-positive bacteria. An introduction of an additional ethyl group worsens the antibacterial activity of 12 in comparison with 11, particularly against E. faecium and S. aureus ATCC 25923. Two propyl groups cause that 13 exhibits even a weaker inhibitory effectivity than 12. These findings are in full agreement with our previous findings concerning N-alkyl and N,N-dialkyl derivatives of diosgenyl 2-amino-2-deoxy-β-D-glucopyranoside [31]. Monoethyl derivatives of both glucosamine and galactosamine are the most active compounds against Gram-positive bacteria in the tested groups, regardless of the fact that hydrochloride of aminogalactoside 5 is completely inactive with respect to the tested bacteria whereas analogous hydrochloride of aminoglucoside is relatively active.
Transformation of the 2-amino group into a 2-ureido group rather negatively influences both antifungal and antibacterial activities of the presented saponins. Among the ureido compounds (8−10) only chloroethylureido derivative 9 exhibits weak inhibitory activity against tested Gram-positive bacteria. Its ethylureido analog 8 is inactive in respect of both fungi and bacteria, likewise phenylureido derivative 10. It would seem that an electronegative Cl atom improves the inhibitory properties of ureido saponins against Gram-positive bacteria. However, the influence of the more electronegative three F atoms on an inhibitory effectivity of trifluoroacetyl derivative 7 is opposite. If N-acetyl derivative 6 is relatively active against tested fungi and bacteria, its N-trifluoroacetyl analog 7 does not act at all. The presented results show how complicated the relationships between structure and biological activity are.
Conclusion
Diosgenyl 2-amino-2-deoxy-β-D-galactopyranoside, as well as its two N-acyl, three 2-ureido and three N-alkyl derivatives are reported. N-Alkylation of the amine function seems to be the most advantageous way of modification. Among the tested compounds, the N-alkyl derivatives are the most active against tested fungi and the N-ethyl derivative exhibits the best inhibitory activity against Gram-positive bacteria. The latter finding is in full agreement with our previous findings concerning the N-ethyl derivative of D-glucosamine. Apart from N-ethyl also N-acetyl and 2-chloroethylureido derivatives exhibit activity against Gram-positive bacteria. The change of D-glucosamine into D-galactosamine in diosgenyl 2-amino-2-deoxy-β-D-glycopyranoside impairs the antimicrobial properties of its hydrochloride.
Supporting Information
Supporting Information File 1: Experimental procedures for the preparation of compounds 1–13, spectroscopic data and information on the method of determination of the minimum inhibitory concentration. | ||
Format: PDF | Size: 341.1 KB | Download |
References
-
Chindo, B. A.; Adzu, B.; Gamaniel, K. S. Saponins: structural diversity, properties and applications. In Saponins: properties, applications and health benefits; Koh, R.; Tay, I., Eds.; Nova Science Publishers Inc.: New York, 2012; pp 1–50.
Return to citation in text: [1] -
Sparg, S. G.; Light, M. E.; van Staden, J. J. Ethnopharmacol. 2004, 94, 219–243. doi:10.1016/j.jep.2004.05.016
Return to citation in text: [1] [2] -
Iorizzi, M.; Lanzotti, V.; Ranalli, G.; De Marino, S.; Zollo, F. J. Agric. Food Chem. 2002, 50, 4310–4316. doi:10.1021/jf0116911
Return to citation in text: [1] -
Zhang, J.-D.; Cao, Y.-B.; Xu, Z.; Sun, H.-H.; An, M.-M.; Yan, L.; Chen, H.-S.; Gao, P.-H.; Wang, Y.; Jia, X.-M.; Jiang, Y.-Y. Biol. Pharm. Bull. 2005, 28, 2211–2215. doi:10.1248/bpb.28.2211
Return to citation in text: [1] -
Yang, C.-R.; Zhang, Y.; Jacob, M. R.; Khan, S. I.; Zhang, Y.-J.; Li, X.-C. Antimicrob. Agents Chemother. 2006, 50, 1710–1714. doi:10.1128/AAC.50.5.1710-1714.2006
Return to citation in text: [1] -
Chapagain, B. P.; Wiesman, Z.; Tsror (Lahkim), L. Ind. Crops Prod. 2007, 26, 109–115. doi:10.1016/j.indcrop.2007.02.005
Return to citation in text: [1] -
Man, S.; Gao, W.; Zhang, Y.; Huang, L.; Liu, C. Fitoterapia 2010, 81, 703–714. doi:10.1016/j.fitote.2010.06.004
Return to citation in text: [1] -
Pérez-Labrada, K.; Brouard, I.; Estévez, S.; Marrero, M. T.; Estévez, F.; Bermejo, J.; Rivera, D. G. Bioorg. Med. Chem. 2012, 20, 2690–2700. doi:10.1016/j.bmc.2012.02.026
Return to citation in text: [1] -
Liu, Z.; Gao, W.; Jing, S.; Zhang, Y.; Man, S.; Wang, Y.; Zhang, J.; Liu, C. J. Ethnopharmacol. 2013, 149, 422–430. doi:10.1016/j.jep.2013.06.033
Return to citation in text: [1] -
Thakur, M.; Melzig, M. F.; Fuchs, H.; Weng, A. Bot.: Targets Ther. 2011, 1, 19–29.
Return to citation in text: [1] -
Yang, C.-R.; Tanaka, O. Advances in Plant Glycosides, Chemistry and Biology; Elsevier: Amsterdam, 1999.
Return to citation in text: [1] [2] -
Hou, S. J.; Zou, C. C.; Zhou, L.; Xu, P.; Yu, D. Q.; Lei, P. S. Chin. Chem. Lett. 2007, 18, 769–772. doi:10.1016/j.cclet.2007.05.007
Return to citation in text: [1] -
Wang, B.; Chun, J.; Liu, Y.; Han, L.; Wang, Y.-s.; Joo, E.-J.; Kim, Y.-S.; Cheng, M.-s. Org. Biomol. Chem. 2012, 10, 8822–8834. doi:10.1039/c2ob26579f
Return to citation in text: [1] -
Bansal, R.; Acharya, P. C. Chem. Rev. 2014, 114, 6986–7005. doi:10.1021/cr4002935
Return to citation in text: [1] -
Fang, M.; Gu, L.; Gu, G.; Fang, J. J. Carbohydr. Chem. 2012, 31, 187–202. doi:10.1080/07328303.2011.639966
Return to citation in text: [1] -
Gu, G.; An, L.; Fang, M.; Guo, Z. Carbohydr. Res. 2014, 383, 21–26. doi:10.1016/j.carres.2013.10.015
Return to citation in text: [1] -
Yang, S.-L.; Liu, X.-K.; Wu, H.; Wang, H.-B.; Qing, C. Steroids 2009, 74, 7–12. doi:10.1016/j.steroids.2008.08.008
Return to citation in text: [1] -
Gao, J.; Li, X.; Gu, G.; Sun, B.; Cui, M.; Ji, M.; Lou, H.-X. Bioorg. Med. Chem. Lett. 2011, 21, 622–627. doi:10.1016/j.bmcl.2010.12.046
Return to citation in text: [1] -
Zhang, Y.; Zhang, Y.-J.; Jacob, M. R.; Li, X.-C.; Yang, C.-R. Phytochemistry 2008, 69, 264–270. doi:10.1016/j.phytochem.2007.06.015
Return to citation in text: [1] -
Yu, B.; Zhang, Y.; Tang, P. Eur. J. Org. Chem. 2007, 5145–5161. doi:10.1002/ejoc.200700452
Return to citation in text: [1] -
Zhao, Y.; An, L.; Gu, G.; Guo, Z. J. Carbohydr. Chem. 2014, 33, 152–168. doi:10.1080/07328303.2014.900564
Return to citation in text: [1] -
Yang, Y.; Laval, S.; Yu, B. Adv. Carbohydr. Chem. Biochem. 2014, 71, 137–226. doi:10.1016/B978-0-12-800128-8.00002-9
Return to citation in text: [1] -
Bednarczyk, D.; Kaca, W.; Myszka, H.; Serwecińska, L.; Smiatacz, Z.; Zaborowski, A. Carbohydr. Res. 2000, 328, 249–252. doi:10.1016/S0008-6215(00)00199-3
Return to citation in text: [1] -
Myszka, H.; Bednarczyk, D.; Najder, M.; Kaca, W. Carbohydr. Res. 2003, 338, 133–141. doi:10.1016/S0008-6215(02)00407-X
Return to citation in text: [1] -
Kaskiw, M. J.; Tassotto, M. L.; Th’ng, J.; Jiang, Z.-H. Bioorg. Med. Chem. 2008, 16, 3209–3217. doi:10.1016/j.bmc.2007.12.022
Return to citation in text: [1] -
Kaskiw, M. J.; Tassotto, M. L.; Mok, M.; Tokar, S. L.; Pycko, R.; Th’ng, J.; Jiang, Z.-H. Bioorg. Med. Chem. 2009, 17, 7670–7679. doi:10.1016/j.bmc.2009.09.046
Return to citation in text: [1] -
Cirioni, O.; Myszka, H.; Dawgul, M.; Ghiselli, R.; Orlando, F.; Silvestri, C.; Brescini, L.; Kamysz, W.; Guerrieri, M.; Giacometti, A. J. Med. Microbiol. 2011, 60, 1337–1343. doi:10.1099/jmm.0.031708-0
Return to citation in text: [1] -
Wang, B.; Liu, Y.; Wang, Y.; Liu, X.; Cheng, M.-S. Bioorg. Med. Chem. Lett. 2012, 22, 7110–7113. doi:10.1016/j.bmcl.2012.09.075
Return to citation in text: [1] -
Fernández-Herrera, M. A.; López-Muñoz, H.; Hernández-Vázquez, J. M. V.; Sánchez-Sánchez, L.; Escobar-Sánchez, M. L.; Pinto, B. M.; Sandoval-Ramírez, J. Eur. J. Med. Chem. 2012, 54, 721–727. doi:10.1016/j.ejmech.2012.06.027
Return to citation in text: [1] -
Bednarczyk, D.; Walczewska, A.; Grzywacz, D.; Sikorski, A.; Liberek, B.; Myszka, H. Carbohydr. Res. 2013, 367, 10–17. doi:10.1016/j.carres.2012.11.020
Return to citation in text: [1] [2] -
Walczewska, A.; Grzywacz, D.; Bednarczyk, D.; Dawgul, M.; Nowacki, A.; Kamysz, W.; Liberek, B.; Myszka, H. Beilstein J. Org. Chem. 2015, 11, 869–874. doi:10.3762/bjoc.11.97
Return to citation in text: [1] [2] [3] [4] -
Jiménez Blanco, J. L.; Ortega-Caballero, F.; Ortiz Mellet, C.; Garcia Fernández, J. M. Beilstein J. Org. Chem. 2010, 6, No. 20. doi:10.3762/bjoc.6.20
Return to citation in text: [1] -
McKay, M. J.; Nguyen, H. M. Carbohydr. Res. 2014, 385, 18–44. doi:10.1016/j.carres.2013.08.007
Return to citation in text: [1] -
Cai, J.; Davison, B. E.; Gianellin, C. R.; Thaisrivongs, S.; Wibley, K. S. Carbohydr. Res. 1997, 300, 109–117. doi:10.1016/S0008-6215(97)00039-6
Return to citation in text: [1] -
Liberek, B.; Melcer, A.; Osuch, A.; Wakieć, R.; Milewski, S.; Wiśniewski, A. Carbohydr. Res. 2005, 340, 1876–1884. doi:10.1016/j.carres.2005.05.013
Return to citation in text: [1]
32. | Jiménez Blanco, J. L.; Ortega-Caballero, F.; Ortiz Mellet, C.; Garcia Fernández, J. M. Beilstein J. Org. Chem. 2010, 6, No. 20. doi:10.3762/bjoc.6.20 |
33. | McKay, M. J.; Nguyen, H. M. Carbohydr. Res. 2014, 385, 18–44. doi:10.1016/j.carres.2013.08.007 |
30. | Bednarczyk, D.; Walczewska, A.; Grzywacz, D.; Sikorski, A.; Liberek, B.; Myszka, H. Carbohydr. Res. 2013, 367, 10–17. doi:10.1016/j.carres.2012.11.020 |
31. | Walczewska, A.; Grzywacz, D.; Bednarczyk, D.; Dawgul, M.; Nowacki, A.; Kamysz, W.; Liberek, B.; Myszka, H. Beilstein J. Org. Chem. 2015, 11, 869–874. doi:10.3762/bjoc.11.97 |
31. | Walczewska, A.; Grzywacz, D.; Bednarczyk, D.; Dawgul, M.; Nowacki, A.; Kamysz, W.; Liberek, B.; Myszka, H. Beilstein J. Org. Chem. 2015, 11, 869–874. doi:10.3762/bjoc.11.97 |
1. | Chindo, B. A.; Adzu, B.; Gamaniel, K. S. Saponins: structural diversity, properties and applications. In Saponins: properties, applications and health benefits; Koh, R.; Tay, I., Eds.; Nova Science Publishers Inc.: New York, 2012; pp 1–50. |
10. | Thakur, M.; Melzig, M. F.; Fuchs, H.; Weng, A. Bot.: Targets Ther. 2011, 1, 19–29. |
23. | Bednarczyk, D.; Kaca, W.; Myszka, H.; Serwecińska, L.; Smiatacz, Z.; Zaborowski, A. Carbohydr. Res. 2000, 328, 249–252. doi:10.1016/S0008-6215(00)00199-3 |
7. | Man, S.; Gao, W.; Zhang, Y.; Huang, L.; Liu, C. Fitoterapia 2010, 81, 703–714. doi:10.1016/j.fitote.2010.06.004 |
8. | Pérez-Labrada, K.; Brouard, I.; Estévez, S.; Marrero, M. T.; Estévez, F.; Bermejo, J.; Rivera, D. G. Bioorg. Med. Chem. 2012, 20, 2690–2700. doi:10.1016/j.bmc.2012.02.026 |
9. | Liu, Z.; Gao, W.; Jing, S.; Zhang, Y.; Man, S.; Wang, Y.; Zhang, J.; Liu, C. J. Ethnopharmacol. 2013, 149, 422–430. doi:10.1016/j.jep.2013.06.033 |
24. | Myszka, H.; Bednarczyk, D.; Najder, M.; Kaca, W. Carbohydr. Res. 2003, 338, 133–141. doi:10.1016/S0008-6215(02)00407-X |
25. | Kaskiw, M. J.; Tassotto, M. L.; Th’ng, J.; Jiang, Z.-H. Bioorg. Med. Chem. 2008, 16, 3209–3217. doi:10.1016/j.bmc.2007.12.022 |
26. | Kaskiw, M. J.; Tassotto, M. L.; Mok, M.; Tokar, S. L.; Pycko, R.; Th’ng, J.; Jiang, Z.-H. Bioorg. Med. Chem. 2009, 17, 7670–7679. doi:10.1016/j.bmc.2009.09.046 |
27. | Cirioni, O.; Myszka, H.; Dawgul, M.; Ghiselli, R.; Orlando, F.; Silvestri, C.; Brescini, L.; Kamysz, W.; Guerrieri, M.; Giacometti, A. J. Med. Microbiol. 2011, 60, 1337–1343. doi:10.1099/jmm.0.031708-0 |
28. | Wang, B.; Liu, Y.; Wang, Y.; Liu, X.; Cheng, M.-S. Bioorg. Med. Chem. Lett. 2012, 22, 7110–7113. doi:10.1016/j.bmcl.2012.09.075 |
29. | Fernández-Herrera, M. A.; López-Muñoz, H.; Hernández-Vázquez, J. M. V.; Sánchez-Sánchez, L.; Escobar-Sánchez, M. L.; Pinto, B. M.; Sandoval-Ramírez, J. Eur. J. Med. Chem. 2012, 54, 721–727. doi:10.1016/j.ejmech.2012.06.027 |
30. | Bednarczyk, D.; Walczewska, A.; Grzywacz, D.; Sikorski, A.; Liberek, B.; Myszka, H. Carbohydr. Res. 2013, 367, 10–17. doi:10.1016/j.carres.2012.11.020 |
31. | Walczewska, A.; Grzywacz, D.; Bednarczyk, D.; Dawgul, M.; Nowacki, A.; Kamysz, W.; Liberek, B.; Myszka, H. Beilstein J. Org. Chem. 2015, 11, 869–874. doi:10.3762/bjoc.11.97 |
3. | Iorizzi, M.; Lanzotti, V.; Ranalli, G.; De Marino, S.; Zollo, F. J. Agric. Food Chem. 2002, 50, 4310–4316. doi:10.1021/jf0116911 |
4. | Zhang, J.-D.; Cao, Y.-B.; Xu, Z.; Sun, H.-H.; An, M.-M.; Yan, L.; Chen, H.-S.; Gao, P.-H.; Wang, Y.; Jia, X.-M.; Jiang, Y.-Y. Biol. Pharm. Bull. 2005, 28, 2211–2215. doi:10.1248/bpb.28.2211 |
5. | Yang, C.-R.; Zhang, Y.; Jacob, M. R.; Khan, S. I.; Zhang, Y.-J.; Li, X.-C. Antimicrob. Agents Chemother. 2006, 50, 1710–1714. doi:10.1128/AAC.50.5.1710-1714.2006 |
6. | Chapagain, B. P.; Wiesman, Z.; Tsror (Lahkim), L. Ind. Crops Prod. 2007, 26, 109–115. doi:10.1016/j.indcrop.2007.02.005 |
19. | Zhang, Y.; Zhang, Y.-J.; Jacob, M. R.; Li, X.-C.; Yang, C.-R. Phytochemistry 2008, 69, 264–270. doi:10.1016/j.phytochem.2007.06.015 |
2. | Sparg, S. G.; Light, M. E.; van Staden, J. J. Ethnopharmacol. 2004, 94, 219–243. doi:10.1016/j.jep.2004.05.016 |
20. | Yu, B.; Zhang, Y.; Tang, P. Eur. J. Org. Chem. 2007, 5145–5161. doi:10.1002/ejoc.200700452 |
21. | Zhao, Y.; An, L.; Gu, G.; Guo, Z. J. Carbohydr. Chem. 2014, 33, 152–168. doi:10.1080/07328303.2014.900564 |
22. | Yang, Y.; Laval, S.; Yu, B. Adv. Carbohydr. Chem. Biochem. 2014, 71, 137–226. doi:10.1016/B978-0-12-800128-8.00002-9 |
15. | Fang, M.; Gu, L.; Gu, G.; Fang, J. J. Carbohydr. Chem. 2012, 31, 187–202. doi:10.1080/07328303.2011.639966 |
17. | Yang, S.-L.; Liu, X.-K.; Wu, H.; Wang, H.-B.; Qing, C. Steroids 2009, 74, 7–12. doi:10.1016/j.steroids.2008.08.008 |
12. | Hou, S. J.; Zou, C. C.; Zhou, L.; Xu, P.; Yu, D. Q.; Lei, P. S. Chin. Chem. Lett. 2007, 18, 769–772. doi:10.1016/j.cclet.2007.05.007 |
13. | Wang, B.; Chun, J.; Liu, Y.; Han, L.; Wang, Y.-s.; Joo, E.-J.; Kim, Y.-S.; Cheng, M.-s. Org. Biomol. Chem. 2012, 10, 8822–8834. doi:10.1039/c2ob26579f |
14. | Bansal, R.; Acharya, P. C. Chem. Rev. 2014, 114, 6986–7005. doi:10.1021/cr4002935 |
18. | Gao, J.; Li, X.; Gu, G.; Sun, B.; Cui, M.; Ji, M.; Lou, H.-X. Bioorg. Med. Chem. Lett. 2011, 21, 622–627. doi:10.1016/j.bmcl.2010.12.046 |
2. | Sparg, S. G.; Light, M. E.; van Staden, J. J. Ethnopharmacol. 2004, 94, 219–243. doi:10.1016/j.jep.2004.05.016 |
11. | Yang, C.-R.; Tanaka, O. Advances in Plant Glycosides, Chemistry and Biology; Elsevier: Amsterdam, 1999. |
34. | Cai, J.; Davison, B. E.; Gianellin, C. R.; Thaisrivongs, S.; Wibley, K. S. Carbohydr. Res. 1997, 300, 109–117. doi:10.1016/S0008-6215(97)00039-6 |
35. | Liberek, B.; Melcer, A.; Osuch, A.; Wakieć, R.; Milewski, S.; Wiśniewski, A. Carbohydr. Res. 2005, 340, 1876–1884. doi:10.1016/j.carres.2005.05.013 |
11. | Yang, C.-R.; Tanaka, O. Advances in Plant Glycosides, Chemistry and Biology; Elsevier: Amsterdam, 1999. |
16. | Gu, G.; An, L.; Fang, M.; Guo, Z. Carbohydr. Res. 2014, 383, 21–26. doi:10.1016/j.carres.2013.10.015 |
31. | Walczewska, A.; Grzywacz, D.; Bednarczyk, D.; Dawgul, M.; Nowacki, A.; Kamysz, W.; Liberek, B.; Myszka, H. Beilstein J. Org. Chem. 2015, 11, 869–874. doi:10.3762/bjoc.11.97 |
© 2017 Myszka et al.; licensee Beilstein-Institut.
This is an Open Access article under the terms of the Creative Commons Attribution License (http://creativecommons.org/licenses/by/4.0), which permits unrestricted use, distribution, and reproduction in any medium, provided the original work is properly cited.
The license is subject to the Beilstein Journal of Organic Chemistry terms and conditions: (http://www.beilstein-journals.org/bjoc)