Abstract
We have prepared a series of new and some literature-reported GABA-amides and determined their effect on the activation of GABAA-receptors expressed in CHO cells. Special attention was paid to the purification of the target compounds to remove even traces of GABA contaminations, which may arise from deprotection steps in the synthesis. GABA-amides were previously reported to be partial, full or superagonists. In our hands these compounds were not able to activate GABAA-receptor channels in whole-cell patch-clamp recordings. New GABA-amides, however, gave moderate activation responses with a clear structure–activity relationship suggesting some of these compounds as promising molecular tools for the functional analysis of GABAA-receptors.
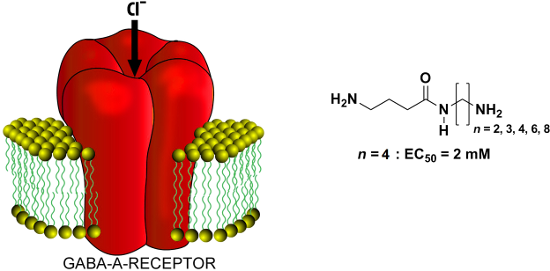
Graphical Abstract
Introduction
γ-Aminobutyric acid (GABA) is the major inhibitory amino acid transmitter of the central nervous system (CNS) of vertebrates (Figure 1).
It plays an important role in a variety of physiological functions, including motion control, vision, relaxation, sleep and many other brain functions [1-3]. In vertebrates GABA activates specific receptors of several classes: GABAA, GABAB and GABAC [4]. GABAA-receptors are transmembrane heterooligomeric proteins, forming chloride (Cl−) selective channels, and are composed of five subunits: two α, two β and one γ subunit [5]. Being highly expressed in the peripheral and central nervous system, GABAA-receptors represent a key therapeutic target for benzodiazepines, barbiturates, neurosteroids and general anesthetics [6-8]. Therefore, in spite of a large variety of existing agonists, antagonists and modulators of GABA-receptors, there is a high interest in the development of new drugs that can interact with these targets. Many compounds of different substance classes are known to modulate the activity of GABAA-receptors [9-12]. One substance group that has been less explored is GABA-amides. Compounds 4a, 4b, 4c, 7a (as triflate salt) and 7c were previously reported to potently activate the GABAA-channels, being "partial, full or superagonists" [13]. However, in their work the authors studied the activity of GABA-amides on GABAA-receptors using chloride-flux assays on synaptoneurosomes. Such preparations can contain many damaged cells leading to a highly variable intracellular Cl− distribution in different cells. In our study we used the patch-clamp technique, which is much more reliable and informative in comparison to the approach based on chloride-flux assays. Like Carlier et al. [13], we noticed that in the course of the GABA-amide synthesis, GABA impurities are generated in the deprotection step. However, in contrast to Carlier et al. [13], who used a modification of Sallers’s procedure [14] (detection limit <0.1 wt %) to check the purity of the compounds, we developed an improved purification procedure and used a more sensitive HPLC–MS analysis to ensure that the GABA-amide products do not contain any detectable amount of GABA (detection limit <0.002 wt %). Our observations may help to give a more complete picture of the ability of GABA-amides to activate GABAA receptors.
Results and Discussion
Boc-Pyrrolidone 1 was nucleophilic-ring-opened by diamines 2a–c or the mono Boc-protected diamines 5a–f in THF (Scheme 1, See Supporting Information File 1 for full experimental data).
Scheme 1: Synthesis of GABA-amide hydrochlorides 4a–c and 6a–f. (i) THF, reflux; (ii) MeOH, HCl (5%); (iii) THF, reflux; (iv) MeOH, HCl (5%).
Scheme 1: Synthesis of GABA-amide hydrochlorides 4a–c and 6a–f. (i) THF, reflux; (ii) MeOH, HCl (5%); (iii) T...
To cleave the Boc-protecting groups, the compounds were dissolved in dilute HCl (5%, EtOH/H2O). It was observed that the use of more concentrated HCl can cause cleavage of the amide bonds and the release of free GABA. To remove any impurities of GABA, which may lead to false test results, all substances were carefully purified: In a first purification step the GABA-amide hydrochlorides were dissolved in MeOH and precipitated by slow addition of Et2O. The precipitates were centrifuged and recrystallized several times from MeOH until no traces of GABA could be detected by HPLC–MS analysis. To determine the sensitivity of the analysis method three stock solutions of GABA were prepared (10−3, 10−5 and 10−7 mol/L) and 1 µL was injected into the HPLC-coupled mass spectrometer. On the basis of the obtained mass spectra, a GABA detection limit of 0.14 pmol (14 pg) was determined.
By examining the Cl− uptake elicited by different GABAA-receptor agonists, Carlier et al. demonstrated that compounds with general structure 4a–c were capable of stimulating Cl− uptake with different efficacy [13]. Moreover, the authors described compound 4b as "superagonist", because it induced a maximum uptake about 50% higher than that achieved by GABA. Surprisingly, the EC50 value for this compound was more than 30-fold higher than for GABA, i.e., at 733 µM and 14.3 µM, respectively. The investigations in this study were performed by using a standard 36Cl−-flux assay in mouse brain synaptoneurosomes. This technique does not allow a comparative analysis on one cell. Moreover, 36Cl−-flux assays were carried out by using a 15 s incubation time. As GABA-receptors usually exhibit strong desensitization at long exposures to agonists, we decided to re-examine these results using patch-clamp recordings and fast perfusion technique for the application of the tested compounds. The activity of synthesized compounds was tested by using CHO cells transiently expressing GABAA-receptors in the configuration (α1-GFP + β2 + γ2Long). To analyze the functional properties of the compounds, we performed monitoring of ionic currents using whole-cell patch-clamp techniques. First, concentration-response curves for GABA were obtained and its EC50 was determined. Then we applied different concentrations of the studied compounds and estimated the minimal concentration that induced currents and, if possible, their EC50. The EC50 for GABA varied in different cells from 4 µM to 15 µM with a mean of 9.5 ± 0.3 µM (x [number of tested cells] = 10) (Figure 2, see Supporting Information File 1 for full experimental data).
![[1860-5397-9-42-2]](/bjoc/content/figures/1860-5397-9-42-2.png?scale=2.0&max-width=1024&background=FFFFFF)
Figure 2: Effect of 7b on GABAA-receptor activation. (A) Superimposed traces of whole-cell currents induced by rapid application of GABA (left) or compound 7b (right) in CHO cells transfected with α1-GFP/β/γ2L combination of GABAA receptor subunits; (B) Concentration dependencies of GABA (closed squares) and 7b (closed circles). EC50s were 12 µM and 10 mM for GABA and 7b, respectively.
Figure 2: Effect of 7b on GABAA-receptor activation. (A) Superimposed traces of whole-cell currents induced b...
Surprisingly, in contrast to previously described observations, our purified compounds 4a–c were not able to activate GABAA-receptors in concentrations up to 10 mM. Figure 3 illustrates this for the compound 4c. Similar results were obtained also for compounds 4a and 4b: on application of 10 mM, the changes in the current were 0 pA, (x = 4 for each compound).
![[1860-5397-9-42-3]](/bjoc/content/figures/1860-5397-9-42-3.png?scale=2.0&max-width=1024&background=FFFFFF)
Figure 3: Absence of GABAA-receptor activation on application of 4c. Top traces: Examples of whole-cell currents on rapid application of 4c on CHO cells transfected with an α1-GFP/β2/γ2L combination of GABAA-receptor subunits. Note that the compound was not able to induce currents even at a concentration of 15 mM.
Figure 3: Absence of GABAA-receptor activation on application of 4c. Top traces: Examples of whole-cell curre...
In contrast, all studied compounds from series 7a–e were capable of inducing ionic currents with different efficacy (Figure 4).
![[1860-5397-9-42-4]](/bjoc/content/figures/1860-5397-9-42-4.png?scale=2.0&max-width=1024&background=FFFFFF)
Figure 4: Variation of EC50 values with the number n of methylene units separating the amide and the ammonium group in compounds 7a–e. Each column represents results from 3–7 cells. The mark [?] on the top of bars indicates that for the EC50‘s for these compounds were not determined precisely.
Figure 4: Variation of EC50 values with the number n of methylene units separating the amide and the ammonium...
Thus, compound 7a (n = 2) activated currents at 2.6 mM with an amplitude of 10–30 pA (x = 4). The compound 7b (n = 3) activated GABA-receptors more strongly and at concentrations of 10 mM induced currents comparable to those for GABA 30–100 µM (Figure 2). The EC50 for compound 7b is 1080 ± 140 µM (x = 4). The efficacy of the compounds is significantly weaker than for GABA. The number of -CH2-units between the amide nitrogen atom and the ammonium moiety of compounds 7 affects the efficacy significantly, reaching a maximum with compound 7c (n = 4). The EC50 for compound 7c is 1750 ± 330 µM (x = 7). Compound 7d (n = 6) also effectively activated GABAA-receptors with an activation threshold of about 100 µM. At concentrations of 10 mM it caused currents similar of those induced by saturated GABA concentrations (30–100 µM) with an EC50 of about 5 mM (Figure 5A–C). The EC50 for compound 7d is 5250 ± 560 µM (x = 6). Kinetics of desensitization and the current–voltage dependencies (Figure 5D) of the studied compounds are similar to those for GABA. Compound 7e (n = 8) weakly activated currents at 5 mM with an amplitude of 10–20 pA (x = 3).
![[1860-5397-9-42-5]](/bjoc/content/figures/1860-5397-9-42-5.png?scale=2.4&max-width=1024&background=FFFFFF)
Figure 5: Effect of 7d on GABAA-receptor activation. (A,B) Superimposed traces of whole-cell currents induced by rapid application of GABA (A) or the compound 7d (B) in CHO cells transfected with an α1-GFP/β2/γ2L combination of GABAA-receptor subunits; (C) Concentration dependencies obtained on application of GABA (red closed square) and 7d (black closed circles). EC50 values were 10.2 µM and 5.1 mM for GABA and 7d, respectively; (D) Current–voltage relations for responses induced by compound 7d. Insert: examples of traces at different membrane potentials. Scales: 2 nA and 1 s.
Figure 5: Effect of 7d on GABAA-receptor activation. (A,B) Superimposed traces of whole-cell currents induced...
Conclusion
Our data suggest that compounds 4a–c are not capable of activating GABAA-receptors. Compounds 7a–e are able to stimulate these receptors and show a distinct structure–activity correlation. The compounds may become useful as molecular tools for the functional analysis of GABAA-receptors.
Supporting Information
Exact synthetic procedures, copies of 1H NMR and HPLC–MS spectra of GABA-amide hydrochlorides 4a–c and 7a–f. Cell culture, transient transfection methods and electrophysical recordings.
Supporting Information File 1: Experimental part. | ||
Format: PDF | Size: 825.9 KB | Download |
Acknowledgements
SB was supported by the European Union Seventh Framework Programme under grant agreement no. HEALTH-F2-2008-202088 ("NeuroCypres" project). α1-GFP was a kind gift from the laboratory of P. Seeburg (Heidelberg, Germany) and β2/γ2Long were kindly provided by Sergeeva OA (Düsseldorf, Germany).
References
-
Sieghart, W. Adv. Pharmacol. (San Diego, CA, U. S.) 2006, 54, 231–263. doi:10.1016/S1054-3589(06)54010-4
Return to citation in text: [1] -
Goetz, T.; Arslan, A.; Wisden, W.; Wulff, P. Prog. Brain Res. 2007, 160, 21–41. doi:10.1016/S0079-6123(06)60003-4
Return to citation in text: [1] -
Olsen, R. W.; Sieghart, W. Neuropharmacology 2009, 56, 141–148. doi:10.1016/j.neuropharm.2008.07.045
Return to citation in text: [1] -
Chebib, M.; Johnston, G. A. R. Clin. Exp. Pharmacol. Physiol. 1999, 26, 937–940. doi:10.1046/j.1440-1681.1999.03151.x
Return to citation in text: [1] -
Olsen, R. W.; Sieghart, W. Pharmacol. Rev. 2008, 60, 243–260. doi:10.1124/pr.108.00505
Return to citation in text: [1] -
Moult, P. R. Biochem. Soc. Trans. 2009, 37, 1317–1322. doi:10.1042/BST0371317
Return to citation in text: [1] -
Bormann, J. Trends Pharmacol. Sci. 2000, 21, 16–19. doi:10.1016/S0165-6147(99)01413-3
Return to citation in text: [1] -
Bowery, N. G. Curr. Opin. Pharmacol. 2006, 6, 37–43. doi:10.1016/j.coph.2005.10.002
Return to citation in text: [1] -
Chebib, M.; Johnston, G. A. R. J. Med. Chem. 2000, 43, 1427–1447. doi:10.1021/jm9904349
Return to citation in text: [1] -
Rudolph, U.; Möhler, H. Annu. Rev. Pharmacol. Toxicol. 2004, 44, 475–498. doi:10.1146/annurev.pharmtox.44.101802.121429
Return to citation in text: [1] -
Möhler, H.; Crestani, F.; Rudolph, U. Curr. Opin. Pharmacol. 2001, 1, 22–25. doi:10.1016/S1471-4892(01)00008-X
Return to citation in text: [1] -
Rudolph, U.; Crestani, F.; Möhler, H. Trends Pharmacol. Sci. 2001, 22, 188–194. doi:10.1016/S0165-6147(00)01646-1
Return to citation in text: [1] -
Carlier, P. R.; Chow, E. S.-H.; Barlow, R. L.; Bloomquist, J. R. Bioorg. Med. Chem. Lett. 2002, 12, 1985–1988. doi:10.1016/S0960-894X(02)00299-8
Return to citation in text: [1] [2] [3] [4] -
Saller, C. F.; Czupryna, M. J. J. Chromatogr. 1989, 487, 167–172. doi:10.1016/S0378-4347(00)83020-0
Return to citation in text: [1]
1. | Sieghart, W. Adv. Pharmacol. (San Diego, CA, U. S.) 2006, 54, 231–263. doi:10.1016/S1054-3589(06)54010-4 |
2. | Goetz, T.; Arslan, A.; Wisden, W.; Wulff, P. Prog. Brain Res. 2007, 160, 21–41. doi:10.1016/S0079-6123(06)60003-4 |
3. | Olsen, R. W.; Sieghart, W. Neuropharmacology 2009, 56, 141–148. doi:10.1016/j.neuropharm.2008.07.045 |
9. | Chebib, M.; Johnston, G. A. R. J. Med. Chem. 2000, 43, 1427–1447. doi:10.1021/jm9904349 |
10. | Rudolph, U.; Möhler, H. Annu. Rev. Pharmacol. Toxicol. 2004, 44, 475–498. doi:10.1146/annurev.pharmtox.44.101802.121429 |
11. | Möhler, H.; Crestani, F.; Rudolph, U. Curr. Opin. Pharmacol. 2001, 1, 22–25. doi:10.1016/S1471-4892(01)00008-X |
12. | Rudolph, U.; Crestani, F.; Möhler, H. Trends Pharmacol. Sci. 2001, 22, 188–194. doi:10.1016/S0165-6147(00)01646-1 |
6. | Moult, P. R. Biochem. Soc. Trans. 2009, 37, 1317–1322. doi:10.1042/BST0371317 |
7. | Bormann, J. Trends Pharmacol. Sci. 2000, 21, 16–19. doi:10.1016/S0165-6147(99)01413-3 |
8. | Bowery, N. G. Curr. Opin. Pharmacol. 2006, 6, 37–43. doi:10.1016/j.coph.2005.10.002 |
5. | Olsen, R. W.; Sieghart, W. Pharmacol. Rev. 2008, 60, 243–260. doi:10.1124/pr.108.00505 |
4. | Chebib, M.; Johnston, G. A. R. Clin. Exp. Pharmacol. Physiol. 1999, 26, 937–940. doi:10.1046/j.1440-1681.1999.03151.x |
14. | Saller, C. F.; Czupryna, M. J. J. Chromatogr. 1989, 487, 167–172. doi:10.1016/S0378-4347(00)83020-0 |
13. | Carlier, P. R.; Chow, E. S.-H.; Barlow, R. L.; Bloomquist, J. R. Bioorg. Med. Chem. Lett. 2002, 12, 1985–1988. doi:10.1016/S0960-894X(02)00299-8 |
13. | Carlier, P. R.; Chow, E. S.-H.; Barlow, R. L.; Bloomquist, J. R. Bioorg. Med. Chem. Lett. 2002, 12, 1985–1988. doi:10.1016/S0960-894X(02)00299-8 |
13. | Carlier, P. R.; Chow, E. S.-H.; Barlow, R. L.; Bloomquist, J. R. Bioorg. Med. Chem. Lett. 2002, 12, 1985–1988. doi:10.1016/S0960-894X(02)00299-8 |
13. | Carlier, P. R.; Chow, E. S.-H.; Barlow, R. L.; Bloomquist, J. R. Bioorg. Med. Chem. Lett. 2002, 12, 1985–1988. doi:10.1016/S0960-894X(02)00299-8 |
© 2013 Raster et al; licensee Beilstein-Institut.
This is an Open Access article under the terms of the Creative Commons Attribution License (http://creativecommons.org/licenses/by/2.0), which permits unrestricted use, distribution, and reproduction in any medium, provided the original work is properly cited.
The license is subject to the Beilstein Journal of Organic Chemistry terms and conditions: (http://www.beilstein-journals.org/bjoc)