Abstract
Most heteroaryl selenides and diselenides are biologically active, with some reported to act as antioxidants and show activities that are medicinally relevant; hence, the development of efficient methods for their synthesis is an important objective. Herein, a simple method for the synthesis of selenides and diselenides bearing imidazo[1,2-a]pyridine rings and their anticancer activity are described. The double C–H selenation of imidazo[1,2-a]pyridine with Se powder was catalyzed by CuI (10 mol %) ligated with 1,10-phenanthroline (10 mol %) at 130 °C under aerobic conditions. The selenides or diselenides were prepared almost selectively using selenium powder in an appropriate quantity under otherwise identical reaction conditions. The prepared selenides and diselenides bearing two imidazo[1,2-a]pyridine rings were all novel compounds. Among the prepared diselenides and selenides that exhibited cytotoxicity against cancer cells, bis[2-(4-methoxyphenyl)imidazo[1,2-a]pyridin-3-yl] diselenide showed an excellent anticancer activity and low cytotoxicity toward noncancer cells, suggesting that this diselenide is a potential lead compound for anticancer therapy.
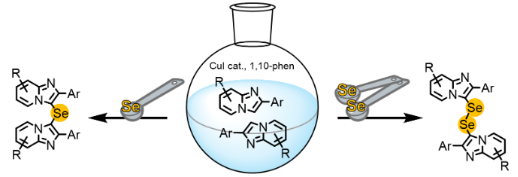
Graphical Abstract
Introduction
The synthesis of organoselenium compounds and their biological activity have attracted considerable attention in research fields directed to drug discovery [1-13]. Among these compounds, heteroaryl selenides and heteroaryl diselenides have been synthesized by a variety of methods [14,15], and many of the targets exhibited biological activity (Figure 1). For example, bis(2-pyridyl) diselenide I has the potential to mitigate oxidative stress and inhibits the AChE activity [16], bis(quinolin-8-yl) diselenide (II) exhibited antioxidant activity in a skin cell model of UVA-induced stress [17], and bis(2-alkylindol-3-yl) selenides III exhibit antitumor activity toward HT-1080 and MG-22A tumor cell lines [18]. The imidazo[1,2-a]pyridine ring is also an important aromatic heterocycle because its derivatives are biologically active and play an important role in medicinal chemistry [19,20]. A number of clinically used drugs and clinical candidates, such as zolpidem, alpidem, saripidem, zolimidine, necopidem, and GSK812397 contain the imidazo[1,2-a]pyridine scaffold [21-26]. 3-(Arylselanyl)imidazo[1,2-a]pyridines IV were reported to act as potential antioxidants and showed antiproliferative activity [27,28]. Consequently, they have attracted much attention, and methods for their syntheses have been actively pursued.
Figure 1: Biologically active selenides and diselenides having heteroaryl groups.
Figure 1: Biologically active selenides and diselenides having heteroaryl groups.
A powerful method for the synthesis of 3-selanylimidazo[1,2-a]pyridines involves the C–H selenation using imidazopyridine and a selenium source in the presence of a transition metal catalyst, such as Cu or Ni [27-32]. In 2011, Zhou et al. reported the pioneering Cu-catalyzed C–H selenation of 2-arylimidazopyridine with diphenyl diselenide in the presence of CuI (10 mol %) [29]. Tandem reactions involving the successive use of imidazopyridine, Se powder, and aryl donors were developed in 2018 as more convenient methods for the synthesis of 3-selanylimidazopyridines. Guo, Li, et al. reacted imidazopyridines, Se powder, and arylboronic acids in the presence of Ag2CO3 (2 equiv) and Cs2CO3 (2 equiv) using a CuI/1,10-phenanthroline catalytic system [28]. Guo, Han, et al. reported a method that used a Cu(OAc)2/1,10-phenanthroline catalyst in the presence of KOH (2 equiv); this method replaced the aryl source from an arylboronic acid with an aryl iodide [30]. Zhou et al. reported the reactions of imidazopyridines, Se powder, and aryl halides in the presence of Na2CO3 (2 equiv) using a NiBr2/2,2-bipyridine system [31]. We also developed reactions of imidazopyridines, Se powder, and triarylbismuthanes using a CuI/1,10-phenanthroline catalytic system that did not require a base or an additive [32]. On the other hand, the synthesis of a diselenide and a selenide bearing two imidazo[1,2-a]pyridine rings, such as bis(imidazo[1,2-a]pyridin-3-yl) selenide and the corresponding diselenides, have only been reported by Paulder et al. [33]; however, the reported reaction was somewhat disadvantageous since it used SeO2 (which is an oxidant) as the selenium source under acidic conditions, and the substrate scope and limitations have not been clarified. Moreover, the syntheses of bis(2-arylimidazo[1,5-a]pyridin-3-yl) selenides and diselenides have recently been investigated using Cu-catalyzed reactions involving imidazo[1,5-a]pyridines, which are isomers of imidazo[1,2-a]pyridines, with Se powder [34]. However, in this reaction, selenides and diselenides were generated in ratios of approximately 1:1; hence, an efficient selective synthesis has not yet been achieved. Inspired by the aforementioned reports and our continuing studies in the synthesis of organoselenium compounds containing imidazo[1,2-a]pyridine rings [27-34], the synthesis of bis(2-arylimidazo[1,2-a]pyridin-3-yl) selenides and diselenides by the Cu-catalyzed tandem C–H selenation of 2-arylimidazo[1,2-a]pyridines with Se powder is presented herein. This reaction was used to selectively synthesize selenides and diselenides bearing two imidazopyridine rings by simply controlling the amount of selenium added. Furthermore, we also report the anticancer activity of the prepared compounds.
Results and Discussion
We previously reported that the Cu-catalyzed C–H selenation of 2-phenylimidazo[1,2-a]pyridine (1a) with 1 equiv of Se powder in the presence of 10 mol % CuI and 1,10-phenanthroline in DMSO at 130 °C under aerobic conditions generated bis(2-phenylimidazo[1,2-a]pyridin-3-yl) diselenide (Table 1, entry 1) [32]. In order to investigate the influence of the amount of Se powder used, 1a was reacted with 0.75 and 0.5 equiv of Se powder under the previously reported optimal experimental conditions. The reaction using 0.75 equiv of Se powder gave the diselenide 2a in 40% yield and the selenide 3a in 58% yield (Table 1, entry 2), while the use of 0.5 equiv of Se powder resulted in the exclusive formation of the selenide 3a in 74% yield (Table 1, entry 3). These result showed that either the selenide or diselenide can be prepared by carefully selecting the amount of selenium powder under otherwise identical reaction conditions.
Table 1: The reaction of 2-phenylimidazopyridine with Se powder.a
|
||||
entry | Se (equiv) | time (h) | yield (%)b | |
2a | 3a | |||
1 | 1 | 1.5 | 86 | – |
2 | 0.75 | 3 | 40 | 58 |
3 | 0.5 | 2 | – | 74 |
a1a: 2.0 mmol. bIsolated yield.
The structures of the diselenide 2a and the selenide 3a were determined by single crystal X-ray diffractometry and are shown in Figure 2. The compounds 2a and 3a both had selenium atoms attached to the 3-positions of their respective imidazo[1,2-a]pyridine rings. The diselenide 2a had a characteristic structure with an exceedingly narrow C–Se–Se–C torsion angle (C1A–Se1A–Se1B–C1B: 49.6(5)°). The imidazo[1,2-a]pyridine planes and the phenyl rings were slightly twisted (C1A–C2A–C3A–C4A: −14.9(19)° and C1B–C2B–C3B–C4B: −21.7(19)°, Figure 2a and Figure 2b). Moreover, the imidazo[1,2-a]pyridine planes and phenyl rings that face each other were almost parallel (mean-interplanar angles: Ph(A)–ImdazoPy(B): 4.59° and Ph(B)–ImdazoPy(A): 4.64°, Figure S2, Supporting Information File 1), and 2a exhibited intramolecular π–π interactions over the whole molecule.
![[1860-5397-16-94-2]](/bjoc/content/figures/1860-5397-16-94-2.png?scale=2.0&max-width=1024&background=FFFFFF)
Figure 2: Ortep drawings of 2a (a and b) and 3a (c and d, thermal elipsoids indicate 50% probability).
Figure 2: Ortep drawings of 2a (a and b) and 3a (c and d, thermal elipsoids indicate 50% probability).
The monoselenide 3a exhibited a C1A–Se–C2A angle of 99.74(6)°, with the two imidazo[1,2-a]pyridine planes oriented orthogonally (Figure 2d). The imidazo[1,2-a]pyridine planes and phenyl rings showed wider torsion angles than those in 2a (C1A–C2A–C3A–C4A: 142.90(14)° and C1B–C2B–C3B–C4B: −43.6(2)°), with possible CH–π interactions between the hydrogen at the 5-position of the imidazo[1,2-a]pyridine unit and the phenyl ring (H1A–C4B: 2.695 Å and H1B–C4A: 3.037 Å).
To demonstrate the scope and limitations of the developed C–H selenation process, the reactions of various imidazopyridines 1 with 1 and 0.5 equiv of Se powder, respectively, were investigated under aerobic conditions in DMSO using the CuI (10 mol %) and 1,10-phenanthroline (10 mol %) catalytic system at 130 °C, the results of which are summarized in Figure 3 and Figure 4. Initially, we examined the syntheses of the diselenides 2 using 1 equiv of Se powder (2.0 mmol) relative to the 2-arylimidazopyridines 1b–i (2.0 mmol). The 2-phenylimidazopyridines 1b–d afforded the corresponding diselenides 2b–d bearing methoxy, methyl, and fluoro substituents at the 6-position of the respective imidazopyridine rings in good to high yield (77–88%, Figure 3). Furthermore, the 2-arylimidazopyridines 1f–h also afforded the corresponding products 2f–h in a yield of 82–89%. The compounds 1e and 1i, bearing a trifluoromethyl group, gave complex mixtures, and the expected products 2e and 2i were not obtained, which suggests that this reaction was sensitive to the electronic nature of the substituent of the imidazopyridine derivative. In addition, the selenide 3 was formed as a minor byproduct, and 2 and 3 were easily separated by silica gel column chromatography (yield of 3b–d, 3f, and 3g, respectively: 6–10%).
Figure 3: The synthesis of bis(2-arylimidazopyridin-3-yl) diselenides. Reaction conditions: 1 (2 mmol), Se (2 mmol), CuI (0.2 mmol), 1,10-phenanthroline (0.2 mmol), DMSO (8 mL), 130 °C, 3 h. The isolated yield is given.
Figure 3: The synthesis of bis(2-arylimidazopyridin-3-yl) diselenides. Reaction conditions: 1 (2 mmol), Se (2...
Figure 4: The synthesis of bis(2-arylimidazopyridin-3-yl) selenides. Reaction conditions: 1 (2 mmol), Se (1 mmol), CuI (0.2 mmol), 1,10-phenanthroline (0.2 mmol), DMSO (8 mL), 130 °C. The isolated yield is given.
Figure 4: The synthesis of bis(2-arylimidazopyridin-3-yl) selenides. Reaction conditions: 1 (2 mmol), Se (1 m...
We next synthesized the selenides 3 using 0.5 equiv of Se powder (Figure 4). The reactions of Se powder with the imidazopyridines 1b–d, f, and h, bearing methoxy, methyl, and fluoro substituents as R1 or R2, gave the corresponding selenides 3b–d, f, and h in good to excellent yield (78–90%). In contrast, the imidazopyridines bearing a trifluoromethyl group gave complex mixtures from which the expected products 3e and 3i were not obtained. The substituents R1 and R2 appeared to influence these reactions in the same manner as they did during the syntheses of the diselenides 2. It is noteworthy that this reaction only gave the selenide 3, with no diselenide byproduct observed.
Control experiments were carried out in order to investigate the reaction mechanism (Scheme 1). Heating the diselenide 2a under the standard conditions gave the selenide 3a in only 14% yield (reaction i, Scheme 1), which suggests that the conversion of 2a into to selenide 3a was difficult following diselenide formation. The reaction of the diselenide 2a with 1 equiv of the imidazopyridine 1a gave the selenide 3a in a high yield (reaction ii, Scheme 1). Moreover, the reaction of the selenide 3a with Se powder afforded the diselenide 2a in 62% yield (reaction iii, Scheme 1). These results show that the diselenide 2a and the selenide 3a are interconvertible in the presence of the imidazopyridine 1a and Se powder. On the other hand, the reaction of the imidazopyridine 1a with 1 equiv of Se powder in the presence of 2 equiv of 1,1-diphenylethylene as a radical scavenger gave the diselenide 2a in a satisfactory yield (78%, reaction iv, Scheme 1): only 2a was isolated in 72% yield even when 0.5 equiv of Se powder was used.
We considered that the reaction mechanism may be similar to the Csp²–H selenation of imidazo[1,5-a]pyridines with Se powder proposed by Murai [34]. Based on this report and the above-mentioned control experiments, a plausible double selenation mechanism is shown in Scheme 2 and Scheme 3. The first step of the reaction involves the generation of the intermediate B via A by the Cu-mediated electrophilic substitution of 1 with selenium. The oxidative homocoupling of the intermediate B then proceeds to give the diselenide 2. On the other hand, in the presence of excess imidazopyridine, the oxidative addition of imidazopyridine 1 to B, followed by the aromatization of C leads to the intermediate D. The intermediate D undergoes a reductive elimination to give the selenide 3, with the generation of Cu(I). The interconversion of the diselenide 2 and the selenide 3 is also possibility in this reaction, with the expected mechanism shown in Scheme 3. The oxidative addition of the copper catalyst to the diselenide 2 generates the intermediate E, which is then attacked by an imidazopyridine 1 at the 3-position to form the intermediate C and the selenide anion F. The intermediate C undergoes a reductive elimination and aromatization to give the selenide 3 and Cu(I). Moreover, the Cu-mediated electrophilic addition of 3 and selenium affords G, which aromatizes to form E and then probably undergoes a reductive elimination to afford the diselenide 2.
The anticancer activity of the novel synthesized bis(2-arylimidazo[1,2-a]pyridin-3-yl) diselenides 2 and selenides 3 was evaluated in human cervical cancer HeLa cells (Figure 5). At 25 µM, each compound significantly decreased the cell viability compared to the control group. When compared, each diselenide 2 was more toxic to HeLa cells than the corresponding selenide 3. Moreover, the 4-methoxyphenyl-containing diselenide 2f and the corresponding selenide 3f exhibited the highest anticancer activity among the diselenides and selenides, respectively. Thus, the 4-methoxyphenyl group appeared to enhance the anticancer activity of the bis(2-arylimidazo[1,2-a]pyridin-3-yl) diselenides and selenides. Furthermore, only the compound 2f showed a higher anticancer activity than doxorubicin (DOX), a well-known anthracycline-based anticancer drug. As shown in Figure 6, 2f also exhibited cytotoxicity against U251 human glioblastoma cells and HKBMM human malignant meningioma cells. Importantly, the diselenide 2f was more cytotoxic toward HeLa cancer cells than to human brain microvascular endothelial (HBME) cells, which are noncancerous (Figure 7). Hence, the diselenide 2f may possibly be highly cytotoxic toward various cancer cell lines and relatively low cytotoxic against noncancer cells.
![[1860-5397-16-94-5]](/bjoc/content/figures/1860-5397-16-94-5.png?scale=2.0&max-width=1024&background=FFFFFF)
Figure 5: The cytotoxic effect of the bis(2-arylimidazo[1,2-a]pyridin-3-yl) diselenides 2 and selenides 3 on human cervical cancer HeLa cells. HeLa cells were exposed with or without each compound at 25 µM for 24 h. The cell viability of HeLa cells was confirmed by MTT assays. The data are represented as mean ± S.D. values of four samples. *p < 0.05; **p < 0.01 compared to the control. DOX: doxorubicin.
Figure 5: The cytotoxic effect of the bis(2-arylimidazo[1,2-a]pyridin-3-yl) diselenides 2 and selenides 3 on ...
![[1860-5397-16-94-6]](/bjoc/content/figures/1860-5397-16-94-6.png?scale=2.0&max-width=1024&background=FFFFFF)
Figure 6: The cytotoxic effect of the bis[2-(4-methoxyphenyl)imidazo[1,2-a]pyridin-3-yl] diselenide 2f on cancer cell lines. Human glioblastoma U251 cells (a) and human malignant meningioma HKBMM cells (b) were exposed to compound 2f for 24 h. The cell viability was confirmed by MTT assays. The data are represented as mean ± SD values of four samples. **p < 0.01 compared to the control (no 2f present).
Figure 6: The cytotoxic effect of the bis[2-(4-methoxyphenyl)imidazo[1,2-a]pyridin-3-yl] diselenide 2f on can...
![[1860-5397-16-94-7]](/bjoc/content/figures/1860-5397-16-94-7.png?scale=2.0&max-width=1024&background=FFFFFF)
Figure 7: Cytotoxic effect of the bis[2-(4-methoxyphenyl)imidazo[1,2-a]pyridin-3-yl] diselenide 2f on a cancer cell line and a noncancer cell line. HeLa cells and human brain microvascular endothelial (HBME) cells were exposed to compound 2f for 48 h. The cell viability was confirmed by MTT assays. The data are represented as mean ± SD values of four samples. Statistical significance when compared to the corresponding HeLa cell group at each concentration point: **p < 0.01.
Figure 7: Cytotoxic effect of the bis[2-(4-methoxyphenyl)imidazo[1,2-a]pyridin-3-yl] diselenide 2f on a cance...
Conclusion
The Cu-catalyzed double C–H selenations of imidazo[1,2-a]pyridines with Se powder afforded novel bis(2-arylimidazopyridin-3-yl) diselenides in satisfactory yields under mild reaction conditions. This reaction also produced the corresponding selenides by simply controlling the amount of Se powder used in the reaction. Among the novel compounds synthesized, the bis[2-(4-methoxyphenyl)imidazo[1,2-a]pyridin-3-yl] diselenide 2f exhibited an excellent anticancer activity toward various cancer cell lines and relatively low cytotoxicity toward noncancer cells, suggesting that the diselenide 2f may potentially be an anticancer therapeutic drug. The application of this reaction to other heterocycles, as well as detailed studies into the C–H selenation and anticancer mechanisms are currently underway in our group.
Supporting Information
Supporting Information File 1: Experimental and cytotoxicity assay details, compound characterization and X-ray data, NMR spectra. | ||
Format: PDF | Size: 2.2 MB | Download |
Supporting Information File 2: Crystal data for 2a. | ||
Format: CIF | Size: 303.7 KB | Download |
Supporting Information File 3: Crystal data for 3a. | ||
Format: CIF | Size: 836.1 KB | Download |
References
-
Wirth, T., Ed. Organoselenium Chemistry; Wiley-VCH: Weinheim, Germany, 2012.
Return to citation in text: [1] -
Ogawa, A. Selenium and tellurium in organic synthesis. In Main Group Metals in Organic Synthesis; Yamamoto, H.; Oshima, K., Eds.; Wiley-VCH: Weinheim, Germany, 2004; Vol. 2, pp 813–866. doi:10.1002/3527602607.ch15
Return to citation in text: [1] -
Santoro, S.; Azeredo, J. B.; Nascimento, V.; Sancineto, L.; Braga, A. L.; Santi, C. RSC Adv. 2014, 4, 31521–31535. doi:10.1039/c4ra04493b
Return to citation in text: [1] -
Manjare, S. T.; Kim, Y.; Churchill, D. G. Acc. Chem. Res. 2014, 47, 2985–2998. doi:10.1021/ar500187v
Return to citation in text: [1] -
Marini, F.; Sternativo, S. Synlett 2013, 24, 11–19. doi:10.1055/s-0032-1317481
Return to citation in text: [1] -
Mukherjee, A. J.; Zade, S. S.; Singh, H. B.; Sunoj, R. B. Chem. Rev. 2010, 110, 4357–4416. doi:10.1021/cr900352j
Return to citation in text: [1] -
Freudendahl, D. M.; Shahzad, S. A.; Wirth, T. Eur. J. Org. Chem. 2009, 1649–1664. doi:10.1002/ejoc.200801171
Return to citation in text: [1] -
Álvarez-Pérez, M.; Ali, W.; Marć, M. A.; Handzlik, J.; Domínguez-Álvarez, E. Molecules 2018, 23, No. 628. doi:10.3390/molecules23030628
Return to citation in text: [1] -
Nogueira, C. W.; Rocha, J. B. T. Arch. Toxicol. 2011, 85, 1313–1359. doi:10.1007/s00204-011-0720-3
Return to citation in text: [1] -
Sanmartín, C.; Plano, D.; Font, M.; Palop, J. A. Curr. Cancer Drug Targets 2011, 11, 496–523. doi:10.2174/156800911795538093
Return to citation in text: [1] -
Sarma, B. K.; Mugesh, G. Org. Biomol. Chem. 2008, 6, 965–974. doi:10.1039/b716239a
Return to citation in text: [1] -
Nogueira, C. W.; Zeni, G.; Rocha, J. B. T. Chem. Rev. 2004, 104, 6255–6286. doi:10.1021/cr0406559
Return to citation in text: [1] -
Mugesh, G.; du Mont, W.-W.; Sies, H. Chem. Rev. 2001, 101, 2125–2180. doi:10.1021/cr000426w
Return to citation in text: [1] -
Ivanova, A.; Arsenyan, P. Coord. Chem. Rev. 2018, 370, 55–68. doi:10.1016/j.ccr.2018.05.015
Return to citation in text: [1] -
Potapov, V. A. Organic diselenides, ditellurides, polyselenides and polytellurides. Synthesis and reactions. In Chemistry of Organic Selenium and Tellurium Compounds; Patai, S.; Rappoport, Z., Eds.; John Wiley & Sons, Ltd.: Chichester, UK, 2013; Vol. 4, pp 765–843. doi:10.1002/9780470682531.pat0716
Return to citation in text: [1] -
Peglow, T. J.; Schumacher, R. F.; Cargnelutti, R.; Reis, A. S.; Luchese, C.; Wilhelm, E. A.; Perin, G. Tetrahedron Lett. 2017, 58, 3734–3738. doi:10.1016/j.tetlet.2017.08.030
Return to citation in text: [1] -
Collins, C. A.; Fry, F. H.; Holme, A. L.; Yiakouvaki, A.; Al-Qenaei, A.; Pourzand, C.; Jacob, C. Org. Biomol. Chem. 2005, 3, 1541–1546. doi:10.1039/b503282m
Return to citation in text: [1] -
Abele, E.; Popelis, J.; Shestakova, I.; Domracheva, I.; Arsenyan, P.; Lukevics, E. Chem. Heterocycl. Compd. 2004, 40, 742–746. doi:10.1023/b:cohc.0000040769.55088.e3
Return to citation in text: [1] -
Enguehard-Gueiffier, C.; Gueiffier, A. Mini-Rev. Med. Chem. 2007, 7, 888–899. doi:10.2174/138955707781662645
Return to citation in text: [1] -
Goel, R.; Luxami, V.; Paul, K. Curr. Top. Med. Chem. 2016, 16, 3590–3616. doi:10.2174/1568026616666160414122644
Return to citation in text: [1] -
Katsura, Y.; Nishino, S.; Takasugi, H. Chem. Pharm. Bull. 1991, 39, 2937–2943. doi:10.1248/cpb.39.2937
Return to citation in text: [1] -
Abe, Y.; Kayakiri, H.; Satoh, S.; Inoue, T.; Sawada, Y.; Inamura, N.; Asano, M.; Aramori, I.; Hatori, C.; Sawai, H.; Oku, T.; Tanaka, H. J. Med. Chem. 1998, 41, 4587–4598. doi:10.1021/jm980330i
Return to citation in text: [1] -
Jain, A. N. J. Med. Chem. 2004, 47, 947–961. doi:10.1021/jm030520f
Return to citation in text: [1] -
Hanson, S. M.; Morlock, E. V.; Satyshur, K. A.; Czajkowski, C. J. Med. Chem. 2008, 51, 7243–7252. doi:10.1021/jm800889m
Return to citation in text: [1] -
Boggs, S.; Elitzin, V. I.; Gudmundsson, K.; Martin, M. T.; Sharp, M. J. Org. Process Res. Dev. 2009, 13, 781–785. doi:10.1021/op9000675
Return to citation in text: [1] -
Linton, A.; Kang, P.; Ornelas, M.; Kephart, S.; Hu, Q.; Pairish, M.; Jiang, Y.; Guo, C. J. Med. Chem. 2011, 54, 7705–7712. doi:10.1021/jm2010942
Return to citation in text: [1] -
Vieira, B. M.; Thurow, S.; da Costa, M.; Casaril, A. M.; Domingues, M.; Schumacher, R. F.; Perin, G.; Alves, D.; Savegnago, L.; Lenardão, E. J. Asian J. Org. Chem. 2017, 6, 1635–1646. doi:10.1002/ajoc.201700339
Return to citation in text: [1] [2] [3] -
Guo, T.; Dong, Z.; Zhang, P.; Xing, W.; Li, L. Tetrahedron Lett. 2018, 59, 2554–2558. doi:10.1016/j.tetlet.2018.05.046
Return to citation in text: [1] [2] [3] [4] -
Li, Z.; Hong, J.; Zhou, X. Tetrahedron 2011, 67, 3690–3697. doi:10.1016/j.tet.2011.03.067
Return to citation in text: [1] [2] [3] -
Guo, T.; Wei, X.-N.; Zhu, Y.-L.; Chen, H.; Han, S.-L.; Ma, Y.-C. Synlett 2018, 29, 1530–1536. doi:10.1055/s-0037-1609758
Return to citation in text: [1] [2] [3] -
Zhu, J.; Zhu, W.; Xie, P.; Pittman, C. U., Jr.; Zhou, A. Tetrahedron 2018, 74, 6569–6576. doi:10.1016/j.tet.2018.09.037
Return to citation in text: [1] [2] [3] -
Kondo, K.; Matsumura, M.; Kanasaki, K.; Murata, Y.; Kakusawa, N.; Yasuike, S. Synthesis 2018, 50, 2200–2210. doi:10.1055/s-0036-1591972
Return to citation in text: [1] [2] [3] [4] -
Hand, E. S.; Paudler, W. W. J. Org. Chem. 1975, 40, 2916–2920. doi:10.1021/jo00908a014
Return to citation in text: [1] [2] -
Shibahara, F.; Kanai, T.; Yamaguchi, E.; Kamei, A.; Yamauchi, T.; Murai, T. Chem. – Asian J. 2014, 9, 237–244. doi:10.1002/asia.201300882
Return to citation in text: [1] [2] [3]
34. | Shibahara, F.; Kanai, T.; Yamaguchi, E.; Kamei, A.; Yamauchi, T.; Murai, T. Chem. – Asian J. 2014, 9, 237–244. doi:10.1002/asia.201300882 |
27. | Vieira, B. M.; Thurow, S.; da Costa, M.; Casaril, A. M.; Domingues, M.; Schumacher, R. F.; Perin, G.; Alves, D.; Savegnago, L.; Lenardão, E. J. Asian J. Org. Chem. 2017, 6, 1635–1646. doi:10.1002/ajoc.201700339 |
28. | Guo, T.; Dong, Z.; Zhang, P.; Xing, W.; Li, L. Tetrahedron Lett. 2018, 59, 2554–2558. doi:10.1016/j.tetlet.2018.05.046 |
29. | Li, Z.; Hong, J.; Zhou, X. Tetrahedron 2011, 67, 3690–3697. doi:10.1016/j.tet.2011.03.067 |
30. | Guo, T.; Wei, X.-N.; Zhu, Y.-L.; Chen, H.; Han, S.-L.; Ma, Y.-C. Synlett 2018, 29, 1530–1536. doi:10.1055/s-0037-1609758 |
31. | Zhu, J.; Zhu, W.; Xie, P.; Pittman, C. U., Jr.; Zhou, A. Tetrahedron 2018, 74, 6569–6576. doi:10.1016/j.tet.2018.09.037 |
32. | Kondo, K.; Matsumura, M.; Kanasaki, K.; Murata, Y.; Kakusawa, N.; Yasuike, S. Synthesis 2018, 50, 2200–2210. doi:10.1055/s-0036-1591972 |
33. | Hand, E. S.; Paudler, W. W. J. Org. Chem. 1975, 40, 2916–2920. doi:10.1021/jo00908a014 |
34. | Shibahara, F.; Kanai, T.; Yamaguchi, E.; Kamei, A.; Yamauchi, T.; Murai, T. Chem. – Asian J. 2014, 9, 237–244. doi:10.1002/asia.201300882 |
32. | Kondo, K.; Matsumura, M.; Kanasaki, K.; Murata, Y.; Kakusawa, N.; Yasuike, S. Synthesis 2018, 50, 2200–2210. doi:10.1055/s-0036-1591972 |
1. | Wirth, T., Ed. Organoselenium Chemistry; Wiley-VCH: Weinheim, Germany, 2012. |
2. | Ogawa, A. Selenium and tellurium in organic synthesis. In Main Group Metals in Organic Synthesis; Yamamoto, H.; Oshima, K., Eds.; Wiley-VCH: Weinheim, Germany, 2004; Vol. 2, pp 813–866. doi:10.1002/3527602607.ch15 |
3. | Santoro, S.; Azeredo, J. B.; Nascimento, V.; Sancineto, L.; Braga, A. L.; Santi, C. RSC Adv. 2014, 4, 31521–31535. doi:10.1039/c4ra04493b |
4. | Manjare, S. T.; Kim, Y.; Churchill, D. G. Acc. Chem. Res. 2014, 47, 2985–2998. doi:10.1021/ar500187v |
5. | Marini, F.; Sternativo, S. Synlett 2013, 24, 11–19. doi:10.1055/s-0032-1317481 |
6. | Mukherjee, A. J.; Zade, S. S.; Singh, H. B.; Sunoj, R. B. Chem. Rev. 2010, 110, 4357–4416. doi:10.1021/cr900352j |
7. | Freudendahl, D. M.; Shahzad, S. A.; Wirth, T. Eur. J. Org. Chem. 2009, 1649–1664. doi:10.1002/ejoc.200801171 |
8. | Álvarez-Pérez, M.; Ali, W.; Marć, M. A.; Handzlik, J.; Domínguez-Álvarez, E. Molecules 2018, 23, No. 628. doi:10.3390/molecules23030628 |
9. | Nogueira, C. W.; Rocha, J. B. T. Arch. Toxicol. 2011, 85, 1313–1359. doi:10.1007/s00204-011-0720-3 |
10. | Sanmartín, C.; Plano, D.; Font, M.; Palop, J. A. Curr. Cancer Drug Targets 2011, 11, 496–523. doi:10.2174/156800911795538093 |
11. | Sarma, B. K.; Mugesh, G. Org. Biomol. Chem. 2008, 6, 965–974. doi:10.1039/b716239a |
12. | Nogueira, C. W.; Zeni, G.; Rocha, J. B. T. Chem. Rev. 2004, 104, 6255–6286. doi:10.1021/cr0406559 |
13. | Mugesh, G.; du Mont, W.-W.; Sies, H. Chem. Rev. 2001, 101, 2125–2180. doi:10.1021/cr000426w |
18. | Abele, E.; Popelis, J.; Shestakova, I.; Domracheva, I.; Arsenyan, P.; Lukevics, E. Chem. Heterocycl. Compd. 2004, 40, 742–746. doi:10.1023/b:cohc.0000040769.55088.e3 |
33. | Hand, E. S.; Paudler, W. W. J. Org. Chem. 1975, 40, 2916–2920. doi:10.1021/jo00908a014 |
17. | Collins, C. A.; Fry, F. H.; Holme, A. L.; Yiakouvaki, A.; Al-Qenaei, A.; Pourzand, C.; Jacob, C. Org. Biomol. Chem. 2005, 3, 1541–1546. doi:10.1039/b503282m |
34. | Shibahara, F.; Kanai, T.; Yamaguchi, E.; Kamei, A.; Yamauchi, T.; Murai, T. Chem. – Asian J. 2014, 9, 237–244. doi:10.1002/asia.201300882 |
16. | Peglow, T. J.; Schumacher, R. F.; Cargnelutti, R.; Reis, A. S.; Luchese, C.; Wilhelm, E. A.; Perin, G. Tetrahedron Lett. 2017, 58, 3734–3738. doi:10.1016/j.tetlet.2017.08.030 |
31. | Zhu, J.; Zhu, W.; Xie, P.; Pittman, C. U., Jr.; Zhou, A. Tetrahedron 2018, 74, 6569–6576. doi:10.1016/j.tet.2018.09.037 |
14. | Ivanova, A.; Arsenyan, P. Coord. Chem. Rev. 2018, 370, 55–68. doi:10.1016/j.ccr.2018.05.015 |
15. | Potapov, V. A. Organic diselenides, ditellurides, polyselenides and polytellurides. Synthesis and reactions. In Chemistry of Organic Selenium and Tellurium Compounds; Patai, S.; Rappoport, Z., Eds.; John Wiley & Sons, Ltd.: Chichester, UK, 2013; Vol. 4, pp 765–843. doi:10.1002/9780470682531.pat0716 |
32. | Kondo, K.; Matsumura, M.; Kanasaki, K.; Murata, Y.; Kakusawa, N.; Yasuike, S. Synthesis 2018, 50, 2200–2210. doi:10.1055/s-0036-1591972 |
27. | Vieira, B. M.; Thurow, S.; da Costa, M.; Casaril, A. M.; Domingues, M.; Schumacher, R. F.; Perin, G.; Alves, D.; Savegnago, L.; Lenardão, E. J. Asian J. Org. Chem. 2017, 6, 1635–1646. doi:10.1002/ajoc.201700339 |
28. | Guo, T.; Dong, Z.; Zhang, P.; Xing, W.; Li, L. Tetrahedron Lett. 2018, 59, 2554–2558. doi:10.1016/j.tetlet.2018.05.046 |
29. | Li, Z.; Hong, J.; Zhou, X. Tetrahedron 2011, 67, 3690–3697. doi:10.1016/j.tet.2011.03.067 |
30. | Guo, T.; Wei, X.-N.; Zhu, Y.-L.; Chen, H.; Han, S.-L.; Ma, Y.-C. Synlett 2018, 29, 1530–1536. doi:10.1055/s-0037-1609758 |
31. | Zhu, J.; Zhu, W.; Xie, P.; Pittman, C. U., Jr.; Zhou, A. Tetrahedron 2018, 74, 6569–6576. doi:10.1016/j.tet.2018.09.037 |
32. | Kondo, K.; Matsumura, M.; Kanasaki, K.; Murata, Y.; Kakusawa, N.; Yasuike, S. Synthesis 2018, 50, 2200–2210. doi:10.1055/s-0036-1591972 |
28. | Guo, T.; Dong, Z.; Zhang, P.; Xing, W.; Li, L. Tetrahedron Lett. 2018, 59, 2554–2558. doi:10.1016/j.tetlet.2018.05.046 |
27. | Vieira, B. M.; Thurow, S.; da Costa, M.; Casaril, A. M.; Domingues, M.; Schumacher, R. F.; Perin, G.; Alves, D.; Savegnago, L.; Lenardão, E. J. Asian J. Org. Chem. 2017, 6, 1635–1646. doi:10.1002/ajoc.201700339 |
28. | Guo, T.; Dong, Z.; Zhang, P.; Xing, W.; Li, L. Tetrahedron Lett. 2018, 59, 2554–2558. doi:10.1016/j.tetlet.2018.05.046 |
30. | Guo, T.; Wei, X.-N.; Zhu, Y.-L.; Chen, H.; Han, S.-L.; Ma, Y.-C. Synlett 2018, 29, 1530–1536. doi:10.1055/s-0037-1609758 |
21. | Katsura, Y.; Nishino, S.; Takasugi, H. Chem. Pharm. Bull. 1991, 39, 2937–2943. doi:10.1248/cpb.39.2937 |
22. | Abe, Y.; Kayakiri, H.; Satoh, S.; Inoue, T.; Sawada, Y.; Inamura, N.; Asano, M.; Aramori, I.; Hatori, C.; Sawai, H.; Oku, T.; Tanaka, H. J. Med. Chem. 1998, 41, 4587–4598. doi:10.1021/jm980330i |
23. | Jain, A. N. J. Med. Chem. 2004, 47, 947–961. doi:10.1021/jm030520f |
24. | Hanson, S. M.; Morlock, E. V.; Satyshur, K. A.; Czajkowski, C. J. Med. Chem. 2008, 51, 7243–7252. doi:10.1021/jm800889m |
25. | Boggs, S.; Elitzin, V. I.; Gudmundsson, K.; Martin, M. T.; Sharp, M. J. Org. Process Res. Dev. 2009, 13, 781–785. doi:10.1021/op9000675 |
26. | Linton, A.; Kang, P.; Ornelas, M.; Kephart, S.; Hu, Q.; Pairish, M.; Jiang, Y.; Guo, C. J. Med. Chem. 2011, 54, 7705–7712. doi:10.1021/jm2010942 |
19. | Enguehard-Gueiffier, C.; Gueiffier, A. Mini-Rev. Med. Chem. 2007, 7, 888–899. doi:10.2174/138955707781662645 |
20. | Goel, R.; Luxami, V.; Paul, K. Curr. Top. Med. Chem. 2016, 16, 3590–3616. doi:10.2174/1568026616666160414122644 |
29. | Li, Z.; Hong, J.; Zhou, X. Tetrahedron 2011, 67, 3690–3697. doi:10.1016/j.tet.2011.03.067 |
© 2020 Matsumura et al.; licensee Beilstein-Institut.
This is an Open Access article under the terms of the Creative Commons Attribution License (http://creativecommons.org/licenses/by/4.0). Please note that the reuse, redistribution and reproduction in particular requires that the authors and source are credited.
The license is subject to the Beilstein Journal of Organic Chemistry terms and conditions: (https://www.beilstein-journals.org/bjoc)